[Source : https://slate.com/technology/2022/08/xenotransplantation-pigs-heart-kidney-donor-virus.html]
How to Raise a Pig for Its Organs
Xenotransplantation could be the future—if only the pigs could be kept virus-free.
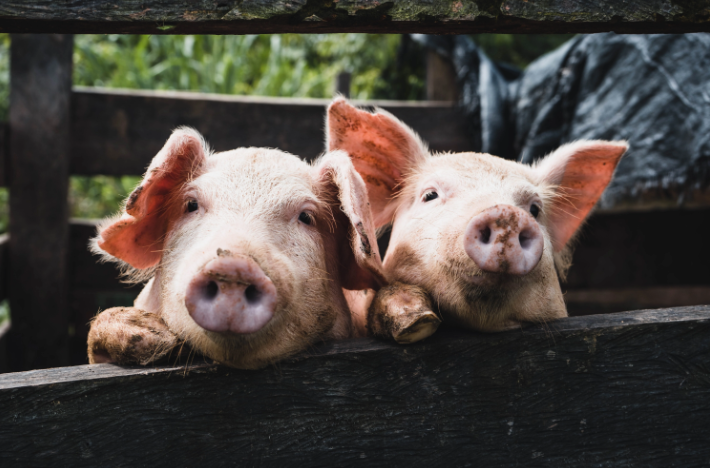
These pigs are too dirty. Photo by Kenneth Schipper Vera on Unsplash
Not too far in the future, everyone will know someone who has a pig organ inside their body. At least, that’s what xenotransplantation researchers hope.
Over the past year, surgeons have been busy creating pig-human hybrids. In New York and Birmingham, they transplanted pig kidneys into three brain-dead humans—proof-of-concept operations. In Baltimore, surgeons transplanted a pig heart into a living person with end-stage heart failure.
If these pig organ transplants became commonplace, they could save a lot of lives. There are over 100,000 Americans on the national transplant list, and seventeen people die every day waiting. Kidneys are highest in demand, followed by livers, hearts, and lungs. The dream is to end this shortage with pigs.
But these transplants aren’t as easy as plucking a pig from a farm and putting its organs into humans. It’s a highly technical process, rife with risks. Take the Baltimore example where the 57-year-old patient David Bennett got infected with the pig virus pCMV and passed away 60 days after his surgery, as reported in The New England Journal of Medicine. Bennett’s death epitomizes the continued danger and uncertainty over xenotransplantation since it’s a process all too easily felled by viruses. There are major hurdles to clear if animals are to become a reliable source of organs for people. Chief among them is how do you make sure the pig’s “donation” won’t be carrying something that infects the recipient?
Non-human primates, such as chimpanzees and baboons, were initially the animal of choice, but they fell out of favor in the ‘90s. Their biggest advantage—similarity to humans—elicited their downfall. Animal rights organizations protested the use of our “closest evolutionary cousins.” And the public was worried that a monkey virus could make it into humans. After all, HIV originated in chimpanzees.
Pigs, in turn, had a lot going for them. With centuries of agricultural experience under our belt, pigs were easier to breed, keep pathogen-free, and even genetically engineer.
That last part is crucial because, before these pigs are even born, they needed to be “humanized” so that their organs are compatible with our bodies. This is done using the gene-editing tool CRISPR. There’s the three-gene pig (as in, three genes have been added or deleted), the five-gene pig, the ten-gene pig, and the sixty-gene pig, with a Goldilocks-like debate over how many changes are just right. Everybody agrees that, at minimum, three pig genes need to be knocked out so that our bodies have a fighting chance of accepting the organ. Beyond that, you have two general theories: “size doesn’t matter” and “the bigger, the better.”
Researchers Joseph Tector and Eckhard Wolf are the minimalists. They believe the limitations of CRISPR call for modesty. For one, CRISPR is not entirely efficient, meaning that it alters only a percentage of earmarked DNA. And secondly, CRISPR causes all kinds of off-target mutations, inadvertently snipping up other bits of DNA. Going for as simple a pig as possible, both argued, is the best way to ensure a consistent product.
Biotechnology companies Revivicor and eGenesis, on the other hand, are the embellishers, hoping that with a more ambitious set of edits, they can eliminate the need for immunosuppression and get the best long-term survival. “Some of our colleagues and competitors are minimum editing,” eGenesis’ CEO Mike Curtis said, “because, well, they can’t do anything else.” After several years of testing and dozens of iterations, Revivicor and eGenesis have been able to ensure more-or-less consistent models.
Regardless of the CRISPR strategy, once the necessary genetic modifications are made, the pig embryos are transferred into a surrogate inside a designated pathogen-free (DPF) facility. The name of the game is to keep everything sterile. Imagine a concrete facility—it’s easy to wash down—with filtered air, UV-treated water, and no way for pigs to go outside. Basically the polar opposite of a pigsty.
For pigs, pregnancy naturally lasts exactly three months, three weeks, and three days. A few days before this 3-3-3 delivery date, surgeons will perform a caesarean section, because vaginal births leave piglets at risk of pCMV and other pathogens. The pigs will go straight from the womb into a bath of disinfectant. Then, they’ll be raised in isolation boxes, away from their mothers.
These pigs are fed a kind of baby formula by technicians dressed up in “spacesuits” (you couldn’t technically go to space in them, but they would comprehensively cover your body.) Regular screenings check for viruses; pigs that test positive for the bad ones—those that might infect humans—are killed. And after about six months when they’ve grown to adult human size, these pigs are ready to have their organs cut out and transplanted—if everything goes well, without any viruses enclosed.
But Bennett, the Baltimore patient who briefly lived with a pig heart, still got pCMV. His pig was screened four separate times before transplantation via nasal swab and PCR, testing negative for the virus every single time. What Bennett’s surgeon Bartley Griffith thinks may have happened is that the pig had a dormant pCMV infection that “hitched a ride” via the donor heart and reactivated in Bennett’s immunosuppressed body. Griffith’s team successfully treated the infection, but it was too late—the virus had likely already done too much damage to Bennett’s heart. “We won the battle, lost the war,” Griffith said.
However, losing this war signals trouble for the pig-organ dream. Every xenotransplantation expert I spoke to was surprised that Bennett was infected with a pig virus. For twenty years, it’s been known that you need to get rid of pCMV to make pig-heart transplants possible, and the protocol for raising pigs has the express purpose of ensuring a clean donor. So, Bennett’s infection by pCMV is not only baffling but also alarming, to say the least. When speaking to The New York Times, Griffith nonetheless predicted, “Knowing it was there, we’ll probably be able to avoid it in future.”
Still, the difficulty of ensuring a clean pig reveals how the road ahead may still be winding and uncertain. We don’t know what pig we should be using. We don’t know whether pig hearts can sustain humans long-term. We don’t know what we don’t know.
After all, if the patient got infected with pCMV of all things, despite the precautions, then, Curtis asked, “what else could possibly happen?”